By Nancy W. Stauffer, MIT Energy Initiative September 17, 2022 Chemical engineers at MIT are utilizing DNA to assist transform CO2 into important chemicals. MIT Assistant Professor Ariel Furst and her coworkers are aiming to DNA to assist direct the procedure. Co2 (CO2) is a substantial item of numerous human activities, consisting of commercial production. It is likewise a significant factor to environment modification. A significant objective in the energy sector has actually been to chemically transform produced CO2 into fuels or other important chemicals. CO2 is offered in abundance, it has actually not yet been commonly utilized to produce value-added items. Why not? Among the primary factors is that CO2 particles are extremely steady. They are not really prone to being chemically transformed to a various type. Researchers have actually looked for products and gadget styles that might assist stimulate that conversion, however absolutely nothing has actually worked all right yet to produce an effective, cost-efficient system. Ariel Furst is the Raymond (1921) and Helen St. Laurent Career Development Professor of Chemical Engineering at MIT. 2 years back, she chose to attempt utilizing something various– a product that gets more attention in conversations of biology than chemical engineering. Currently, arises from operate in her laboratory show that her uncommon method is settling. Teacher Ariel Furst (center), undergraduate Rachel Ahlmark (left), postdoc Gang Fan (right), and their coworkers are using biological products, consisting of DNA, to attain the conversion of co2 to important items. Credit: Gretchen Ertl The stumbling blockChallenges start with the initial step in the CO2 conversion procedure. CO2 needs to be chemically transformed into carbon monoxide gas (CO) prior to being changed into a helpful item. Electrochemistry, a procedure in which input voltage supplies the additional energy required to make the steady CO2 particles respond, can promote that conversion. The issue is that accomplishing the CO2-to-CO conversion needs big energy inputs– and even then, CO comprises just a little portion of the items that are produced. To check out chances for enhancing this procedure, Furst and her research study group concentrated on the electrocatalyst, a product that boosts the rate of a chain reaction without being consumed at the same time. The driver is essential to effective operation. Inside an electrochemical gadget, the driver is frequently suspended in a liquid (water-based) service. When an electrical capacity (basically a voltage) is used to an immersed electrode, liquified CO2 will– assisted by the driver– be transformed to CO. There’s one stumbling block: The driver and the CO2 need to satisfy on the surface area of the electrode for the response to take place. In some research studies, the driver is distributed in the service, however that technique needs more driver and isn’t extremely effective, according to Furst. “You need to both await the diffusion of CO2 to the driver and for the driver to reach the electrode prior to the response can take place,” she describes. As an outcome, scientists worldwide have actually been checking out various techniques of “immobilizing” the driver on the electrode. Linking the driver and the electrodeBefore Furst might explore that obstacle, she required to choose which of the 2 kinds of CO2 conversion drivers to deal with: the conventional solid-state driver or a driver comprised of little particles. In taking a look at the literature, she concluded that small-molecule drivers held the most assure. While their conversion performance tends to be lower than that of solid-state variations, molecular drivers use one essential benefit: They can be tuned to stress responses and items of interest. 2 techniques are frequently utilized to incapacitate small-molecule drivers on an electrode. One includes connecting the driver to the electrode by strong covalent bonds– a kind of bond in which atoms share electrons; the outcome is a strong, basically irreversible connection. The other establish a non-covalent accessory in between the driver and the electrode; unlike a covalent bond, this connection can quickly be broken. Neither technique is perfect. In the previous case, the driver and electrode are securely connected, making sure effective responses; however when the activity of the driver deteriorates with time (which it will), the electrode can no longer be accessed. In the latter case, an abject driver can be gotten rid of; however the specific positioning of the little particles of the driver on the electrode can’t be managed, resulting in an irregular, frequently reducing, catalytic performance– and merely increasing the quantity of driver on the electrode surface area without issue for where the particles are positioned does not fix the issue. What was required was a method to place the small-molecule driver securely and precisely on the electrode and after that launch it when it breaks down. For that job, Furst relied on what she and her group consider a sort of “programmable molecular Velcro”: deoxyribonucleic acid, or DNA. Including DNA to the mixMention DNA to the majority of people, and they think about biological functions in living things. The members of Furst’s laboratory view DNA as more than simply hereditary code. “DNA has these truly cool physical homes as a biomaterial that individuals do not frequently consider,” she states. “DNA can be utilized as a molecular Velcro that can stick things together with extremely high accuracy.” Furst understood that DNA series had actually formerly been utilized to incapacitate particles on surface areas for other functions. She designed a strategy to utilize DNA to direct the immobilization of drivers for CO2 conversion. Her method depends upon a well-understood habits of DNA called hybridization. The familiar DNA structure is a double helix that forms when 2 complementary hairs link. When the series of bases (the 4 foundation of DNA) in the specific hairs compare, hydrogen bonds form in between complementary bases, securely connecting the hairs together. Utilizing that habits for driver immobilization includes 2 actions. The researchers connect a single hair of DNA to the electrode. They connect a complementary hair to the driver that is drifting in the liquid option. When the latter hair comes up to the previous, the 2 hairs hybridize; they end up being connected by numerous hydrogen bonds in between appropriately paired bases. As an outcome, the driver is strongly attached to the electrode by methods of 2 interlocked, self-assembled DNA hairs, one linked to the electrode and the other to the driver. Much better still, the 2 hairs can be removed from one another. “The connection is steady, however if we warm it up, we can get rid of the secondary hair that has the driver on it,” states Furst. “So we can de-hybridize it. That enables us to recycle our electrode surface areas– without needing to take apart the gadget or do any severe chemical actions.” Speculative investigationTo check out that concept, Furst and her group– postdocs Gang Fan and Thomas Gill, previous college student Nathan Corbin PhD ’21, and previous postdoc Amruta Karbelkar– carried out a series of experiments utilizing 3 small-molecule drivers based upon porphyrins, a group of substances that are biologically essential for procedures varying from enzyme activity to oxygen transportation. 2 of the drivers include an artificial porphyrin plus a metal center of either cobalt or iron. The 3rd driver is hemin, a natural porphyrin substance utilized to deal with porphyria, a set of conditions that can impact the nerve system. “So even the small-molecule drivers we picked are type of motivated by nature,” remarks Furst. In their experiments, the private investigators initially required to customize single hairs of DNA and deposit them on among the electrodes immersed in the service inside their electrochemical cell. This sounds simple, it did need some brand-new chemistry. Led by Karbelkar and third-year undergraduate scientist Rachel Ahlmark, the group established a quick, simple method to connect DNA to electrodes. For this work, the researchers’ focus was on connecting DNA, however the “tethering” chemistry they established can likewise be utilized to connect enzymes (protein drivers), and Furst thinks it will be extremely beneficial as a basic technique for customizing carbon electrodes. When the single hairs of DNA were transferred on the electrode, the scientists manufactured complementary hairs and connected to them among the 3 drivers. When the DNA hairs with the driver were contributed to the service in the electrochemical cell, they easily hybridized with the DNA hairs on the electrode. After half-an-hour, the scientists used a voltage to the electrode to chemically transform CO2 liquified in the option and utilized a gas chromatograph to examine the makeup of the gases produced by the conversion. The group discovered that when the DNA-linked drivers were easily distributed in the service, they were extremely soluble– even when they consisted of small-molecule drivers that do not liquify in water by themselves. While porphyrin-based drivers in service typically stick together, as soon as the DNA hairs were connected, that disadvantageous habits was no longer obvious. The DNA-linked drivers in service were likewise more steady than their unmodified equivalents. They didn’t deteriorate at voltages which triggered the unmodified drivers to deteriorate. “So simply connecting that single hair of DNA to the driver in service makes those drivers more steady,” states Furst. “We do not even need to put them on the electrode surface area to see enhanced stability.” When transforming CO2 in this method, a steady driver will offer a constant existing in time. Speculative outcomes revealed that including the DNA avoided the driver from deteriorating at voltages of interest for useful gadgets. With all 3 drivers in option, the DNA adjustment considerably increased the production of CO per minute. Permitting the DNA-linked driver to hybridize with the DNA linked to the electrode brought even more enhancements, even compared to the very same DNA-linked driver in service. As an outcome of the DNA-directed assembly, the driver ended up securely connected to the electrode, and the driver stability was even more boosted. In spite of being extremely soluble in liquid options, the DNA-linked driver particles stayed hybridized at the surface area of the electrode, even under severe speculative conditions. Incapacitating the DNA-linked driver on the electrode likewise substantially increased the rate of CO production. In a series of experiments, the scientists kept track of the CO production rate with each of their drivers in service without connected DNA hairs– the standard setup– and after that with them paralyzed by DNA on the electrode. With all 3 drivers, the quantity of CO created per minute was far greater when the DNA-linked driver was debilitated on the electrode. Furthermore, incapacitating the DNA-linked driver on the electrode significantly increased the “selectivity” in regards to the items. One consistent difficulty in utilizing CO2 to produce CO in liquid options is that there is an inescapable competitors in between the development of CO and the development of hydrogen. That propensity was reduced by including DNA to the driver in option– and much more so when the driver was paralyzed on the electrode utilizing DNA. For both the cobalt-porphyrin driver and the hemin-based driver, the development of CO relative to hydrogen was substantially greater with the DNA-linked driver on the electrode than in option. With the iron-porphyrin driver they had to do with the exact same. “With the iron, it does not matter whether it’s in option or on the electrode,” Furst discusses. “Both of them have selectivity for CO, so that’s excellent, too.” Development and plansFurst and her group have actually now shown that their DNA-based technique integrates the benefits of the conventional solid-state drivers and the more recent small-molecule ones. In their experiments, they attained the extremely effective chemical conversion of CO2 to CO and likewise had the ability to manage the mix of items formed. And they think that their method needs to show scalable: DNA is economical and commonly readily available, and the quantity of driver needed is numerous orders of magnitude lower when it’s paralyzed utilizing DNA. Based upon her work so far, Furst assumes that the structure and spacing of the little particles on the electrode might straight affect both catalytic performance and item selectivity. Utilizing DNA to manage the exact positioning of her small-molecule drivers, she prepares to examine those effects and after that theorize style criteria that can be used to other classes of energy-conversion drivers. Eventually, she wishes to establish a predictive algorithm that scientists can utilize as they develop electrocatalytic systems for a wide array of applications. Referral: “DNA-based immobilization for enhanced electrochemical co2 decrease” by Gang Fan, Nathan Corbin, Thomas Gill, Amruta Karbelkar and Ariel Furst, 19 May 2022, ChemRxiv. DOI: 10.26434/ chemrxiv-2022- qll2k This research study was supported by a grant from the MIT Energy Initiative Seed Fund.
Read More
Utilizing DNA To Convert Carbon Dioxide Into Valuable Products
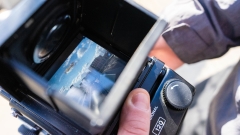